A study, published in the journal Nature, shows newly developed microscopic robots that will be able to unclog arteries, deliver drugs, and perform incisions.
The robots’ engineering is inspired on bacteria, as they are soft and flexible. Scientists can manipulate their movements through electromagnetic fields, making them swim in a particular direction and performing specific tasks.
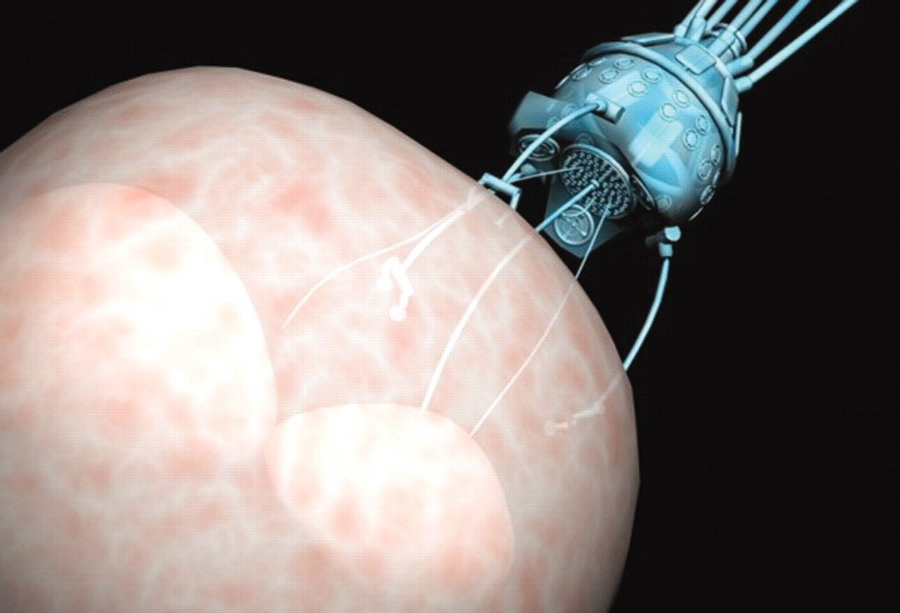
Hen-Wei Huang led the study on microrobots resembling eukaryotic cells, from ETH Zurich’s Institute for Robotics and Intelligent Systems, alongside EPFL scientist Selman Sakar.
Robots inspired on real cells
The goal was to develop microscopic machines that were able to navigate in confined spaces, while also being able to perform medical operations without being deemed as invasive or unsafe. Because the resulting microrobots are so small, they are still considered to be lackluster regarding how many functions they can perform and how well can they maneuver when exposed to a biological environment.
The microrobots were inspired by origami, as they can fold in different ways to control how they swim in their field of operation as they receive commands through electromagnetic fields.
The resulting robots are flexible and lack conventional motorized structures. This is because they are made out of a biocompatible hydrogel embedded with magnetic nanoparticles, which can change the robot’s shape and trajectory.
One of the inspiring microorganisms behind the project was the bacterium responsible for African trypanosomiasis, or ‘sleeping sickness’. It is a bacterium that has a tail for propulsion to move along heterogeneous environments, but as it enters its hosts’ bloodstream, the tail is hidden to preserve the bacterium’s integrity and promote survival. The developed microrobots were able to perform a similar process just as they perceived increase in temperature, in this case with a laser. When it senses heat, the robot wraps its tail around its body.
“Our new production method lets us test an array of shapes and combinations to obtain the best motion capability for a given task. Our research also provides valuable insight into how bacteria move inside the human body and adapt to changes in their microenvironment,” explained Selman Sakar.
He stressed the necessity of the microrobots being completely safe for the patient’s body, as it is possible that the immune system recognizes them as a threat.
Creating thermosensitive robotic bacteria, a bioengineering feat
One of the challenges was to design a soft nanocomposite matrix to determine how the building blocks of the microrobot will bend, depending on the commands emitted through electromagnetic waves. Bending the robot’s body was more complex on its boundaries, as the interconnected parts of the layer are more easily manipulated than the parts that lie on the edge of each layer that compose the robot. Several bending models were created to efficiently store the flagellum.
The research team had to develop a unique folding technique which was able to accommodate the robot’s tail into a helix around its body, while only receiving electromagnetic waves as stimuli. In the end, the folding technique based itself solely on the behavior of the edge sections of the robot, thus being able to follow a magnetic axis and then transform itself into a helix, wrapping the flagellum around the newly formed cylindrical body. In the end, the particles had to be oriented in perpendicular lines, rather than just being scattered along the superficial layer of the robot.
When the robot’s hydrogel becomes exposed to temperatures of 40°C, it expands into a flat layer. As the temperature increases beyond 42°C, it morphs into a tubular shape. Anything beyond 45°C transforms the robot into a deformed cylinder.
The research team managed to make it, so the morphing process is reversible, so the microrobots can go back to their original shape whenever temperature decreases accordingly. It is possible to change the temperature threshold for the process by changing the type of hydrogel comprising the structure of the robot. By removing a single polymer of the hydrogel, the team managed to change the temperature threshold from 45 to 32°C.
Through tests using lasers, it was possible to heat the machines, so they achieved their final form after 65 seconds. Then, they were allowed to cool down and turned back into their original shape after 74 seconds.
Because each component of the microrobot was able to be engineered independently, it allowed for an ample room for improvement regarding the robot’s propulsion process. The results were fascinating, as the robots moved just like the cells that inspired them would. The following image shows some tests of the robot’s mobility capabilities depending on its current form.
The next step in robotics is to allow them to perceive stimuli through light, temperature and anything that could be manipulated by humans to send a message or to originate a response. Recently, a similar project yielded a tiny soft robotic ray fish which was able to respond to light. The researchers managed to steer the ray fish robot through an obstacle course.
Its movement was dictated by the different light patterns emitted from the outside, as its photosensitive internal structure acted accordingly. The team that developed the photosensitive ray fish robot compared it’s performance to that a human heart.
Source: Nature